Learning
Article curated by Ginny Smith
From the moment we're born, we begin to learn about the world around us, and this continues for the rest of our lives. But exactly how does learning happen in the brain? And how does it change throughout out lives? With reference to other animals and how they seem to learn, scientists are working hard to find out the answers.
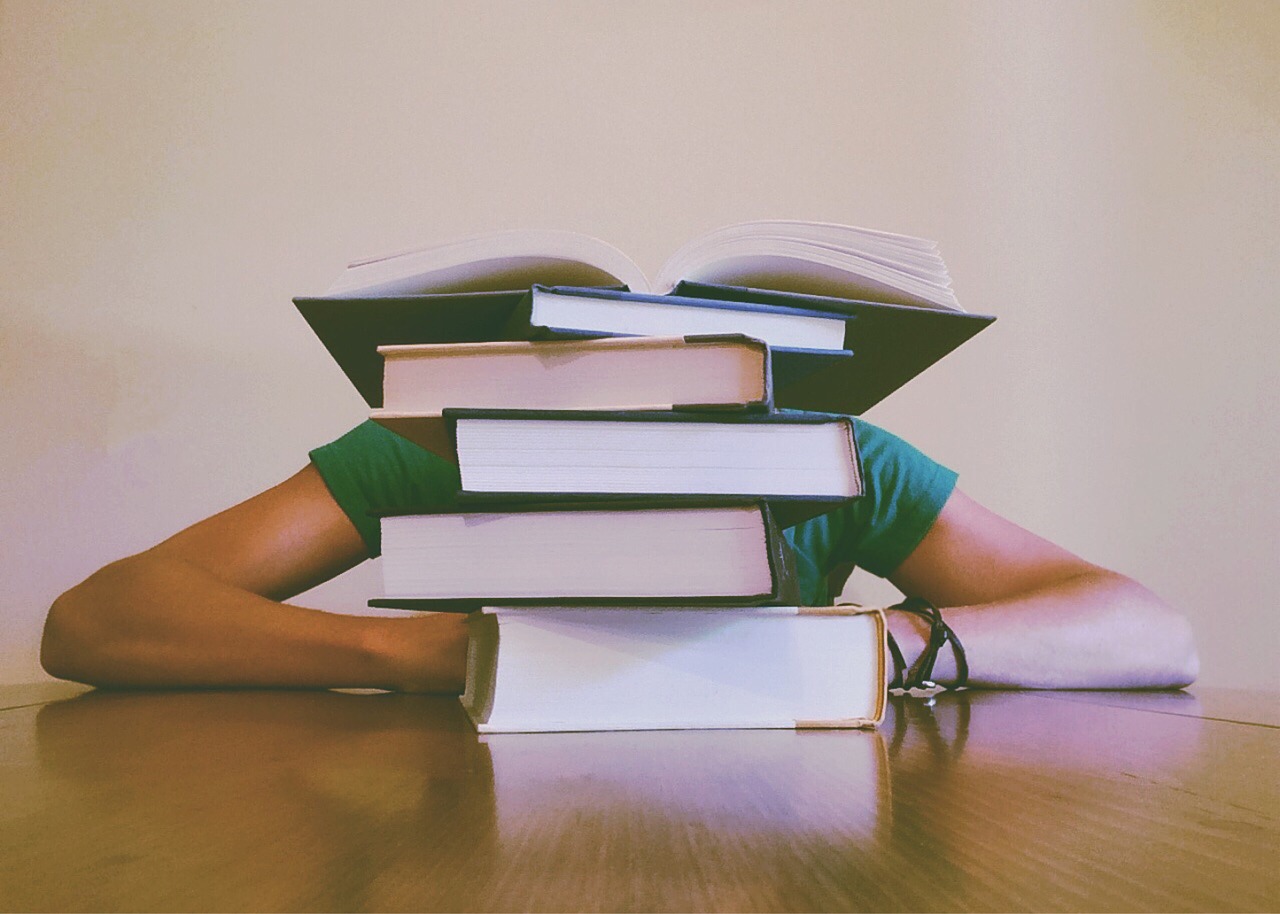
Learning in the brain
When we learn, our brains change: some pathways become stronger and others weaker. This ability to change is known as plasticity, and the ease with which it happens varies throughout our lives. When we are young, our brains are more plastic to enable us to learn rapidly but, as we age, efficiency and stability become more important and our brains become less flexible. However, we don't know exactly what causes these changes, or why they happen at different times for different abilities. If it were possible to reverse changes, and put the adult brain back into learning mode, this could be hugely beneficial, not just for general learning (who hasn't wished they could pick up a language as easily as a child?), but also for anyone who has suffered a stroke or other form of brain damage[1].
Learn more about Rewiring the Brain.



In order to enter long term storage, memories must be consolidated. This process begins within minutes of learning, but continues for up to a few weeks. Exactly what is happening in this process isn’t fully understood, but, for many years, scientists thought that the hippocampus repeatedly activates regions of the cortex, strengthening the connections there and effectively transferring the memory from the hippocampus to the cortex, where it can be stored permanently. However, a recent study in mice suggests that the hippocampal memory and the cortex version are created at the same time, but that the cortical memory isn't used for the first few days after it is formed – the memory is immature or silent. If connections between the hippocampus and cortex are blocked, the memory never matures. If the hippocampus is damaged, the ability to store long term memories can even be completely lost.
Learn more about Memory in the brain.



Despite not knowing exactly how it works, it seems humans are getting better at learning, and more intelligent, although there may be more to this finding that meets the eye.
IQ tests are designed to keep the population average at 100; but this means the tests have to get harder and harder – by about 1 point every 3 years. That may not sound much, but it would mean half of the world population from the time of WW1 would be classed as "mentally disabled" by modern standards! Clearly, something is wrong.
Various theories exist have attempted to explain why. Could it be education? On average, we stay in school longer, and maybe we are taught in a different way – less rote learning and more abstract thinking, which makes us better suited to the IQ tests than our parents and grandparents. Or are we simply better at sitting exams? Another idea is that better nutrition is responsible for the rise. Prenatal nutrition affects birth weight, and birth weight is correlated to higher IQs. One study also found that iodine defficiency in pregnant mothers is linked to lower IQ scores in their children.
James Flynn, who the effect is named after, doesn't think we are any more intelligent than our ancestors.It's like a weightlifter and swimmer. They may have the same muscles when they were fertilised in the womb, but they would have different muscles at autopsy, wouldn't they?
he said, in an interview with the BBC So today at autopsy, certain portions of our brain, for example those which use logic and abstraction, would have been exercised more and look differently. Other portions of the brain would have shrivelled a bit.

Learning through the lifespan
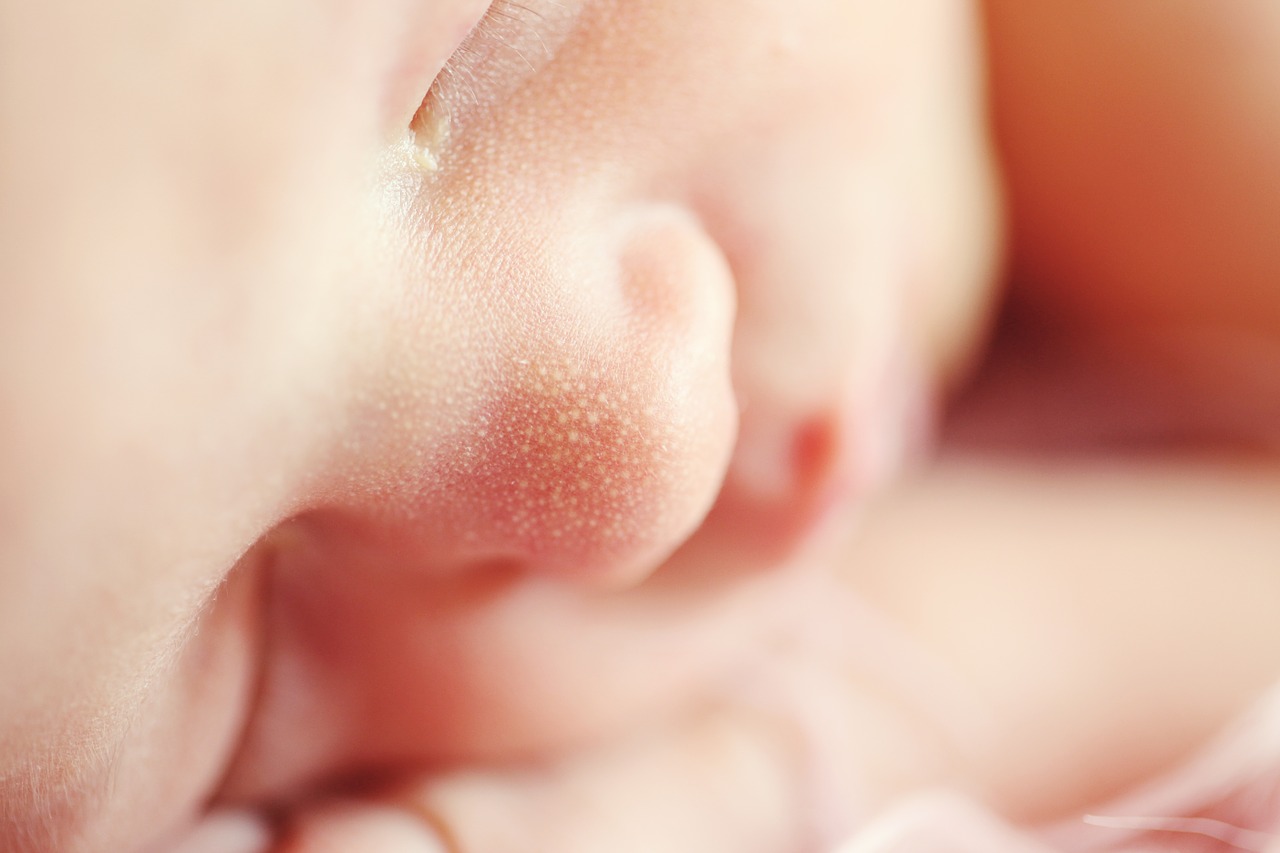
From the moment they are born, babies start learning about the world around them. But how can we find out what they know? Most psychological studies involve instructions, questions and answers. But you can’t ask a newborn baby to press a button, answer a question, or rate something on a scale.
One thing many newborn babies can do, and enjoy doing, is looking at things. So one common way of finding out what babies like is to give them two options and see which one they choose to look at. Using this technique, we now know that babies are born with a preference for faces. Even just a few hours after birth, they will choose to look at a face, or something that resembles a face, over almost any other stimuli.
There is still debate over whether babies are innately drawn specifically to faces, or whether they are drawn to various low-level properties that faces have, such as high contrast and top-heavy shape. Whatever it is, over our first few months of life, we become experts in recognising and categorising faces, and this practice continues throughout our lives.


These looking time experiments can be taken one step further. If you show a baby the same image over and over again, they will get bored and stop looking at it. If you then show them something new, they will look longer again. This can be used to work out what they can discriminate. If, for example, they get bored of a red square, but look longer at a red circle, you know they can tell squares are different from circles. If a green square continued to bore them, you would know they couldn’t distinguish red from green.
Some scientists believe this technique can be used to understand infants' conceptual knowledge. If, for example, they look longer at an impossible event, like an object teleporting, they are said to be surprised by that event, and therefore understand that it shouldn't happen. Research suggests babies understand physical concepts including: solidity (they are surprised when an object is able to pass through another object), occlusion (that objects don't disappear when they are blocked from view), and containment (a big object can't fit into a smaller one).
Of course, there are all sorts of concerns about the validity of this method, and it has led to some strange finding, with babies supposedly understanding concepts that children don’t seem to grasp until much later when you ask them directly. For example, babies won't reach for a hidden object until 8 months, but looking time experiments suggest they know it's there. So why don't they reach? More research is definitely needed.


Now, most scientists talk about sensitive periods rather than critical periods – times when our brains are more ready to absorb new information. This doesn’t mean we can’t do it at other times, but during these sensitive periods learning is easier and faster.
Learn more about whether humans have critical periods for learning.



As they grow up, it becomes a little easier to work out what children have learnt about the world they live in. One ability that takes a while to grasp is understanding how other people think and feel – this is known as Theory of Mind (ToM). Typically, children can pass ToM tests around the age of 4-5. The most common is the Sally-Ann test, which is usually acted out for the child using puppets. The story goes: Sally and Ann are friends. Sally has a marble. She puts in in her box then leaves the room. Ann comes in and moves the marble from the box to the basket. She leaves. Sally comes back in, and wants her marble. Where does she look? Young children will say she looks in the basket, while older children can understand her false belief – that she thinks it is in the box. But how this understanding develops isn't known. Is it simply that 4-5 years of learning are needed to grasp ToM? Or is there something in the brain that changes to allow this ability to develop? It’s the age old question; nature or nurture. And the answer is that we don’t know for sure – and there is no ethical way to find out, because we can’t keep a child in isolation for 5 years to see if their brain differs from other children's. Another complicating factor is that looking time tasks have shown infants are surprised when Sally looks in the wrong place (where the marble is rather than where she left it) at less than 2 years of age! So could it be that they know, on some level, where she ought to look, but can't articulate it? It could be that rather than the development of ToM, we're seeing the development of inhibition, and under 4s can't inhibit their own knowledge so as to give the right answer. Another complication is that the pass age changes depending on the difficulty of the test. At around 2 years, children can understand intentions, and when something is done by accident rather than on purpose. Conversely the ability to recognise a social faux-pas that could cause embarrassment doesn't develop until around the age of 9. Also, young children fail on similar tasks which don't involve a false belief. An example of this would be identifying where an object was when a photo was taken of the scene, when it has been moved since. All this means we are a long way from understanding exactly when and how children develop ToM.


Play is thought to be vital for children to learn about the physical and social world around them, but we don't know what it is about playing that is so important. We see play across the animal kingdom in more intelligent and social animals. This suggests it has an evolutionary advantage, but not for simpler animals like fruit flies. Exactly what this advantage is is not known – it could equally be that it is only more intelligent animals that have time to play, or that extended childhoods allow it.
Learn more about why do we play?.



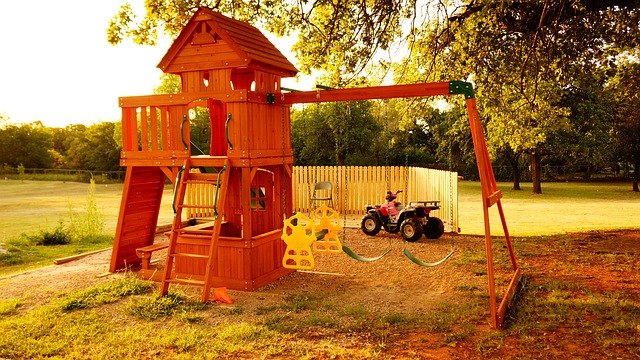

If playing video games can actually help cognitive development, surely games actually designed for the purpose would be even better? If you could improve your memory, attention and reaction time just by playing a few simple games for 15 minutes a day, wouldn’t you want to? Brain training games are marketing this promise – but does it work?
A review published in the journal Psychological Science in the Public Interest suggests the evidence for their benefit is weak[3]. Researchers analysed 374 studies of brain training – all the literature cited by the leading brain training companies. They found that the majority of studies didn’t measure up to the best practice they had defined and weren’t good science. The problems found ranged from small sample sizes to a lack of control group or baseline analysis to compare the post-training results to. Without this, any findings are meaningless. Many studies also failed to account for the placebo effect – if you are told that playing a game will make you better at something, you may get better at it just because you expect to, with no help from the game at all. With all these problems, most of the studies just can’t be relied upon.
There were a few good studies amongst those reviewed, but they didn't show that video game brain training helped improve brain function. Proponents often liken the brain to a muscle, and say that by exercising it, like we do a muscle, we can keep it working well. But this analogy is flawed: if you start lifting weights at the gym, working on your upper body strength, you can rightly expect this exercise to provide benefits in your everyday life – making it easier for you to carry heavy shopping bags or helping you to swim faster. But imagine if that didn’t happen. All the work you put in at the gym meant you were able to lift heavier and heavier dumbbells while you were there, but when you tried to lift something different, or use the muscle in another way, couldn't. This is what seems to be happening with brain training games. People who use them do become better at the specific games they are practising, but they don't see general benefits.
So is there anything you can do to ensure your brain stays healthy? Luckily, the answer is yes – exercise. There is a lot of evidence that physical exercise has benefits for the brain as well as the body, as does a healthy diet and an active social life. So if you want to stave off the declines of ageing, you might want to put down the video games and sign up to that social running club!
Learn more about /aging.



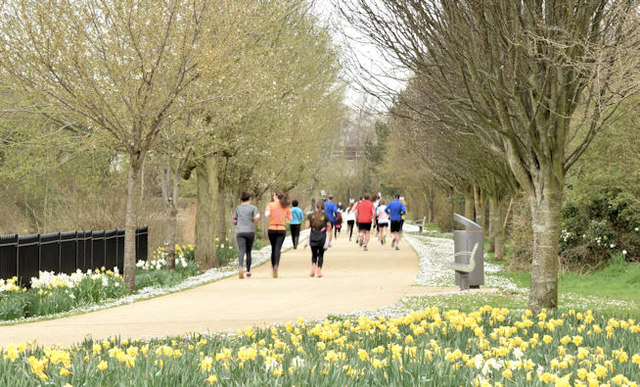


Boost your learning

Prebiotics provide nutrients for intrinsic gut bacteria and make the metabolism more efficient. Some evidence suggests they can also sharpen your mind, and scientists have suggested that they might impact children’s progress in school, and are looking to test whether this is so.



Animal Learning
Learning is crucial for surival, and can be seen even in very simple animals like slime mould. These amazing creatures can be acellular: containing multiple nuclei inside one cell, like an amoeba. As they grow, they spread out in all directions before retracting the branches that don't find food and strengthening those that do. This allows them to learn where food is, and even to map the most efficient route through a maze, or recreate the Tokyo subway! Even more amazingly, slime moulds can learn to anticipate events. In one study, researchers blasted them with cold air at regular intervals, slowing their growth. After a while, they began to slow down just before the time when the next blast of air would be administered. They don't have anything resembling a brain, so nothing in the mould should be able to store a memory, meaning the mystery remains; how do they learn?

Machine learning
Can we teach machines to learn?
Researchers have been attempting to teach machines to learn images using artificial neural networks (ANNs), a family of statistical learning models inspired by biological neural networks. A network consists of 10-30 stacked layers of artificial neurones. When an input stimulus, such as an image, is provided, the first layer will communicate with the subsequent layers until the final layer is reached and it generates an output. Knowing how each layer analyses the image differently is challenging, but the idea is that each layer focusses on a progressively higher abstract aspect of the image. To test their results, researchers asked the machines to output images, and found the AI was able to generate a picture of a banana: a weird, fuzzy, banana, but a recognisable one.
Even though these processes are based on well-known mathematical models, we understand very little about why they work, and why certain others don't. This ability to visualise images and produce them from noise has been dubbed “dreaming”.
Scientists are also interested in the role emotions play in learning. Is it different to feel emotions rather than to recognise and understand them? They are now employing learning techniques such as Q-learning and other model-free machine learning processes to probe reward-based learning that mimics human emotions.
In the “mAIry’s Room” thought experiment, mAIry is confined to a grey room from birth and accesses the outside only through a black and white monitor. She is a brilliant scientist and has learnt all there is to learn about colour and perception. One day she experiences purple for the first time. The question asked by philosopher is, has she learnt anything? mAIry doesn't gain any extra explicit knowledge, but her brain has done something new in its processing.
This is known as TD-Lambda (temporal difference) learning: learning by the difference between a predicted reward and the actual reward. Where learning is the goal, AI can treat certain inputs as “special” or an “experience”, valuing them beyond their informational value. These inputs are thus AI “values”. This then allows smart AI to hold evolving values or ideas about what is “right”.
Learn more about /emotions.



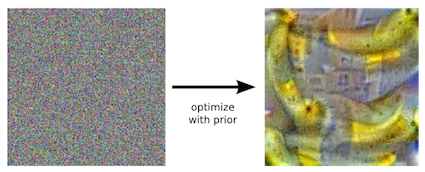


Machine learning can be used in all kinds of fields. For example, new predictive models have been created using machine learning and random forest classification to identify how proteins bind to engineered nanomaterials in biological and environmental systems[4]. This work could have implications in pollution remediation and drug delivery.



Another research area that may benefit from machine learning is making organic crystals. Although researchers now have banks and banks of data on what will and what will not crystallise, there is no simple relationship, and a huge processing demand to try to unravel it. However, researchers at Oxford University including Richard Cooper are currently investigating the use of AI to better predict which crystals will and will not form from organic molecules. AI employs machine learning tactics to look for statistical patterns across large data sets and make predictions that expand on the set. So far, results have looked promising.
Learn more about /artificial intelligence.


This article was written by the Things We Don’t Know editorial team, with contributions from Ed Trollope, Ginny Smith, Cait Percy, Rowena Fletcher-Wood, and Holly Godwin.
This article was first published on 2017-06-02 and was last updated on 2021-07-23.
References
why don’t all references have links?
[1] Vogel, G., (2012). Can We Make Our Brains More Plastic? Science 338(6103):36-39. doi: 10.1126/science.338.6103.36.
[2] Miller, G., (2012). How Are Memories Retrieved? Science 338(6103):30-31. doi: 10.1126/science.338.6103.30-b.
[3] Simons, D,J., et al., (2016). Do Brain-Training Programs Work? Psychological Science in the Public Interest 17(3):103-186. doi: 10.1177/1529100616661983.
[4] Findlay, Matthew R., et al. Machine learning provides predictive analysis into silver nanoparticle protein corona formation from physicochemical properties. Environmental Science: Nano 5.1 (2018): 64-71.
Recent learning News
Get customised news updates on your homepage by subscribing to articles