Cosmic microwave background
Article curated by Grace Mason-Jarrett
In 1965, a soft hiss was detected by the radio telescopes of two astronomers in the Bell Laboratories... a hiss so faint that previous technologies had been unable to detect it, despite it seemingly coming from every point in the sky. This enigmatic hum was named the cosmic microwave background (CMB), and we now recognise it as very low energy electromagnetic radiation (microwaves) filling every part of the universe, perhaps left over from the Big Bang. While the CMB may be omnipresent, the faint signal we are able to detect makes it very difficult to accurately observe it. Now, thanks to highly sensitive instruments placed on satellites orbiting our planet, we have an extensive map of the cosmic microwave background covering the entire sky. This map shows all its fluctuations and other details. By analysing it, scientists can find patterns that may provide clues about the early universe, and detect unexpected phenomena, that open up new questions!
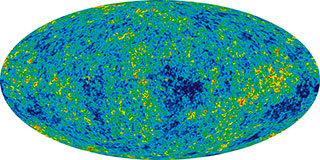
The cosmic microwave background radiation is the afterglow of the Big Bang, a fossil record of the radiation from the very early universe, an image of the moment only a few hundred thousand years into the life of the universe when everything had cooled enough for atoms to form. When these atoms formed, there were far fewer unattached charged particles around for light to interact with, allowing it to propagate freely, and the universe ceased being opaque to light. By using very sensitive detectors, scientists are able to measure variations in the faint level of this fossil microwave radiation across the entire sky. In the universe before this point, for normal matter, the electromagnetic force would have played a far more important role than gravity because it is so much stronger, but should have cancelled out across the whole universe. However, measurements show small but significant local variations which are understood to be the source of the structure in the universe today. Without these small areas of material varying in density compared to it's surroundings, we would be unable to explain how the material in the universe had grouped together to form large structures like galaxies or galaxy clusters and the massive, near empty voids in between them. But the question is... how did these variations form?



Gravitational waves



However, the more they look into it, the more gravitational anomalies astronomers find, including:
– Extra fast stars, which move faster than they should according to observed distributions of normal matter.
– The flyby anomaly, where spacecraft receive greater than predicted acceleration during gravity assist manouevres.
– The observed increasing rate of expansion of space.
– Planetary orbits widening faster than expected if their sole cause is the sun losing mass by radiating energy.
– Photons from the cosmic radiation background gaining more energy than expected when travelling through space, suggesting gravity falls off faster than usual at certain distance scales.
– Extra massive hydrogen clouds – where hydrogen clumps together more than expected.
– The weakness of gravity.
There's more about these in our article on gravity.
Learn more about /gravity.


Galactic centre microwave haze
The European Space Agency’s Planck satellite has added even more detail to our image of the CMB, revealing an unusual haze surrounding the centre of our galaxy. At first glance, this blurry bubble appeared to be very similar to the type of energy (known as synchrotron emission) astronomers usually associate with supernova events. The difference is, this cloud detected by Planck is brighter at different frequencies, and so supernovae cannot be to blame.
Other guesses include galactic winds or even elusive dark matter particles interacting with each other. Once the source of this mysterious fog is solved, astronomers can focus on looking at the CMB without the galactic haze interfering with results.

Fluctuations in the CMB density give us a snap-shot of an early universe made up of individual hydrogen atoms whizzing around, too fast and hot to combine with each other. But we don't know for sure: we've so far found no signals from after the CMB formed. Any light emitted was snapped up by the hydrogen smog! Telescopes simply cannot ‘see’ through the mist and so this era is known as The Dark Ages.
Theories suggest that over time the hydrogen slowed down and collected to form nebulae from which the very first stars formed. Thermal energy from these stars may have caused atoms to lose electrons, or pick up extra ones – ionising the universe. This is known as the Epoch of Re-ionisation, during which light from every part of the spectrum reappeared. Astronomers are still looking for light from the first stars formed and signals from hydrogen atoms during The Dark Ages, which could unveil invaluable information about how our universe came to be the way it is now.

Dark matter


Polarisation of the CMB
Imagine light travelling as a wave along a string. It can vibrate in any direction: up and down, side to side, forwards and backwards... This is known as unpolarised, and this is how light starts out. Polarised light only vibrates in one direction, and when we detect it, we learn something about the material it's travelled through.
Astronomers have now noticed swirls and arches tracing out intricate patterns in the polarisation of the CMB.
Some theories say that the fingerprint-esque pattern could be a result of Einstein’s gravitational waves – ripples transporting gravitational energy throughout spacetime. If this is true, it could evidence Inflation Theory – the theory that the universe expanded at an explosive speed in an infinitesimally small amount of time shortly after the Big Bang. However, measurements of the polarisation were taken by a ground based telescope known as BICEP2[1], while assuming that the galactic haze and other foreground radiation such as large clouds of carbon monoxide in our galaxy is negligible – a fairly big assumption when we don't yet know what the causes of these are. Furthermore, the polarisation may not have been caused by gravitational waves at all, and may simply be a result of the radiation interacting with space dust[2].
Learn more about Cosmic Microwave Background Polarization.


Inflation theory
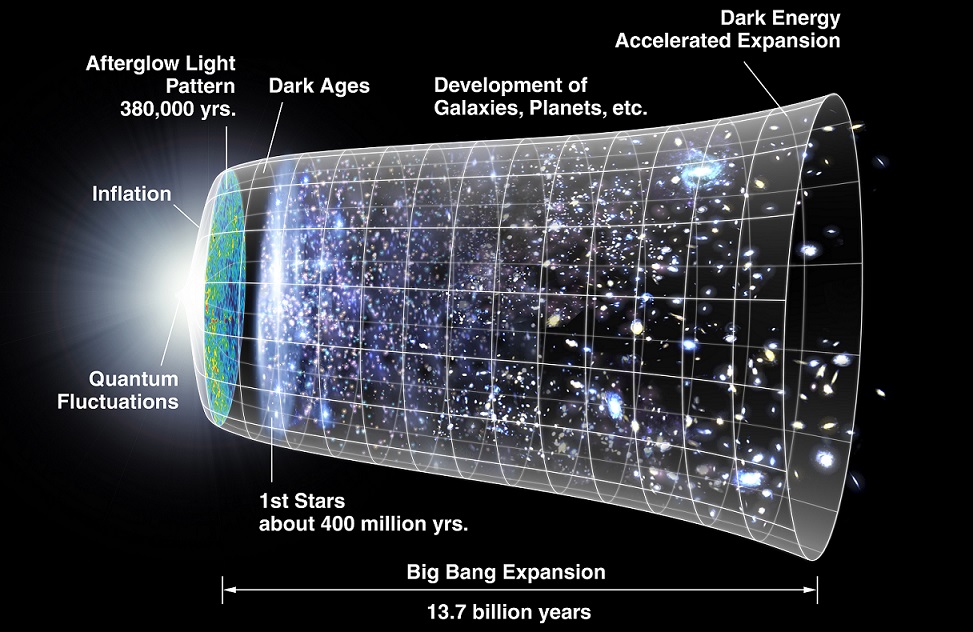
Our model of the early history of the universe suggests that it cooled as it expanded following the Big Bang. This cooling process may have triggered changes in the way in which the universe behaved, just like a liquid freezing into a solid (these changes are known as phase transitions). These phase transitions probably didn't happen simultaneously throughout the universe, and should have left behind cosmic textures. However, so far we haven't found these textures in the cosmic microwave background – scientists suggest they may just be too faint, and we need more sensitive instruments to detect them.


One alternative theory to Inflation Theory suggests that light rays in early universe travelled faster than the speed of light today. They propose that this happens at extreme temperatures, and estimate the spectral index of the universe to be 0.96478. Our current measurement is 0.968, and as estimates improve, whether the practical number moves towards or away from 0.96478 should tell us whether this theory is likely.



Black holes may also hold clues as to the formation of the early universe... but did they form as the first stars did, or did the first stars form the first black holes? There are currently a number of NASA affiliated missions seeking out answers to questions surrounding the evolution of the universe: – SOFIA (2014) – Hubble(1990) – Spitzer(2003) – Herschel (2009-2013) – JWST (2018)


From gravitational waves to dark matter to theories of the early universe, it seems the impact of the cosmic microwave background (CMB) is as wide as the background is. A fingerprint of our history, it provides us with so much information, and we are still untangling it – and looking for more, because there is also some information missing!
This article was written by the Things We Don’t Know editorial team, with contributions from DLR, Jon Cheyne, Cait Percy, Grace Mason-Jarrett, and Rowena Fletcher-Wood.
This article was first published on 2015-08-27 and was last updated on 2021-07-06.
References
why don’t all references have links?
[1] Planck takes Magnetic Fingerprint of our Galaxy ESA Science & Technology.
[2] Flauger, R., Hill, J. C., & Spergel, D. N. (2014). Toward an understanding of foreground emission in the BICEP2 region. Journal of Cosmology and Astroparticle Physics, 2014(08), 039.
Recent cosmic microwave background News
Get customised news updates on your homepage by subscribing to articles